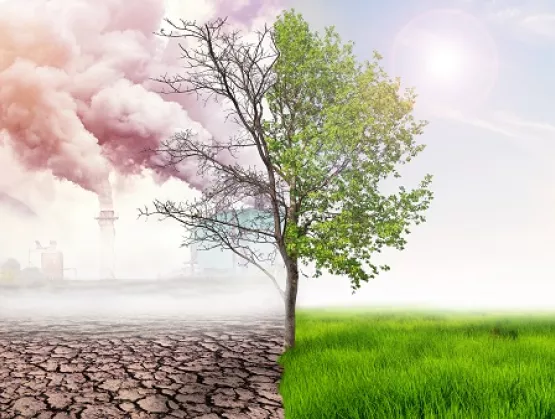
Why do we need environmental air pollution sensors?
Today we need environmental air pollution sensors more than ever to ensure that we have clean and safe outdoor and indoor air. Although federal rules have improved air pollution over the past several decades, more than 110 million Americans still live in counties where air quality is below national standards. An estimated 100,000 Americans die prematurely each year of illnesses caused or exacerbated by polluted air.
“Cars and trucks are much cleaner than they were, power plants are cleaner, industrial operations are cleaner,” said Paul Billings, Senior Vice President Advocacy for the American Lung Association. But cleaner air is not clean air.”
While scientists have long known that air pollution may exacerbate asthma and other respiratory illnesses, new data suggests polluted air leads to higher COVID-19 higher death rates and brain inflammation that can contribute to dementia and autism.
To understand the importance of air quality and how we can apply existing sensors and develop new ones, we look both outdoors and indoors (see Figure 1). Outdoor air quality relates to gaseous and particulate pollutants, defined by the Air Quality Index (AQI). The AQI became a standard based on regional thresholds for a set of key outdoor pollutants: four gaseous pollutants (sulfur dioxide, nitrogen dioxide, carbon monoxide, ozone) and particulates (PMs) of different sizes such as < 10 μm (PM10) and < 2.5 μm (PM2.5). At present, the AQI is measured using traditional analytical instruments. Despite their high acquisition and maintenance costs, these instruments are the only solution to accurately measure these pollutants in the presence of variable environmental background.
Figure 1. Examples of outdoor and indoor air quality markers
Indoor air quality (IAQ) is also of growing concern. Formaldehyde, benzene, carbon monoxide, and carbon dioxide are some of the key pollutants with restricted concentration levels in residential, office and industrial buildings. Sources of these and other gaseous pollutants include building materials and equipment, workplace cleansers, and building occupants. Regulatory agencies and building occupants use different methodologies to estimate IAQ using gaseous and particulate pollutant analyzers. These estimates also consider air humidity and temperature that affect indoor air quality.
Where are we today with environmental sensors?
The top three requirements for modern gas sensors include:
- the sensor reliability to provide accurate readings in diverse environmental conditions over desired period of use
- low power, to extend battery life or to eliminate its need, and
- low cost, to facilitate their ubiquitous deployments.
Advances in electronics, microfabrication, and packaging have delivered recent important developments in reducing the power consumption and miniature packaged solutions. Recent R&D efforts are also increasing the number of successful gas sensor field deployments for outdoor and indoor air quality monitoring. Figure 2 illustrates three examples of recent developments in gas sensors that meet requirements of diverse customers.
Electrochemical sensors from SPEC Sensors were collocated with EPA instruments for monitoring of NO2 and O3 in Chicago’s Array of Things Project. Figure 2A shows that these new cost-effective sensors track well the EPA instruments. Advancements in circuit quality, sampling, enclosure design, and initial calibration/compensation were all essential in achieving these results. While this example clearly demonstrates the usability of these sensors in this particular application, the expectations that low-cost, off-the-shelf sensors will match the performance of EPA reference systems that cost 50x-100x more must be adjusted.
- A micropackaged sensor suite from Bosch Sensortec includes sensors for total volatile organic compounds (TVOCs), temperature, humidity, and pressure. TVOC measurements are needed according to the guidelines by the German Federal Environmental Agency. To report TVOC, the sensor algorithm tracks the TVOC-related resistance of the metal oxide sensor, corrects sensor resistance for ambient temperature and humidity, and outputs the TVOCs Index of Air Quality between 0 (clean air) and 500 (heavily polluted air) as shown in Figure 2B.
- A recent GE-developed dielectric excitation scheme of metal oxide sensing materials provided a highly desired and long-awaited calibration stability of sensors for monitoring of fugitive methane gas emissions in all-weather conditions. These sensors were used in several field validation campaigns in Oklahoma, North Dakota, Arkansas, and British Columbia and had stable performance after more than 400 days, as compared to an initial calibration (see Figure 2C). Such stable sensor performance has become possible by switching from the conventional resistive mode of operation of metal oxide sensing elements to the dielectric excitation scheme.
Figure 2. Examples of applications of contemporary gas sensors based on different detection principles.
(A) Outdoor performance of NO2 and O3 electrochemical sensors versus EPA-validated instruments.
(B) Calibration results of a BME680 metal oxide gas chemiresistor upon exposures to TVOCs (blue stair-profile) and its ± 15% confidence interval band as the Index of Air Quality.
(C) Calibration stability of a sensor with an innovative dielectric excitation scheme implemented for monitoring of fugitive methane gas emissions after multiple uses in diverse field validation campaigns.
Key challenges and solutions toward realizing new applications
In this era of data-on-demand, environmental sensors could enable countless new applications. Imagine you have a gas sensor conveniently integrated into a smartphone or a watch. You are commuting to work, and your sensor alerts you that the subway station through which you are traveling has very poor air quality. How might this alert affect your behavior? Would you put on a mask, change your commuting route to a twice-longer one, or petition the city? What if you are attending a parade downtown with your asthmatic child, and your device informs you that the air is clean? Would you skip the parade if you knew that your sensor was only 10% accurate? How would you avoid a risk of ending with your asthmatic child in a hospital?
Design principles of modern sensors originate in the 20th century for detection of high gas levels from leaks, but they did not anticipate the applications proposed now. By design, existing sensors have only a single output – e.g. resistance, voltage, current, light intensity – that mathematically cannot correct for the sensor instabilities caused by the complex chemical background and variable temperature and humidity conditions. Thus, often these simple sensors perform best when pollution levels are high and when the compound of interest swamps others. As a practical example, there are dozens of gaseous pollutants in ambient air with their toxicity that differ 1,000-10,000 fold. Often, the insufficient reliability and accuracy of existing sensors in the field conditions is a significant bottleneck toward the broad adoption of gas sensors.
According to the United States Environmental Protection Agency (EPA), the correlation between readings of low-cost sensors versus reference monitors varies widely from 1% to 80%. The EPA also states that no low-cost sensors meet Regulatory Monitoring requirements, and the World Meteorological Organization emphasizes that “low-cost sensors are not currently a direct substitute for reference instruments, especially for mandatory purposes.” However, we now have the increasing number of examples of reliable operation in complex environments (Figure 2) in addition to important advances in reduced power and size of contemporary sensors. Still, the key challenges to realize new applications are often the lack of required accuracy and reliability of available sensors for new contemplated applications.
Is it possible to offer low-cost sensors for at least some applications and some gases with the degree of accuracy approaching more expensive specialized instruments? We, the SEMI-MSIG Device Working Group, are saying: Yes. To deliver on this bold statement, our SEMI community brings new technological solutions to the 100-year old general design of gas sensors.
Our next blog What is in the Air will provide details on our activities of SEMI-MSIG Device Working Group to establish standards and new measurement schemes to reduce effects from uncontrolled ambient conditions and to improve stability, limit of detection, and dynamic range of environmental sensors. Also learn how new MSIG members can impact this important working group.
The MEMS & Sensors Industry Group (MSIG) is a SEMI technology community that enables the MEMS and sensor industry to address common challenges, innovate and accelerate business results.
Radislav A. Potyrailo is Principal Scientist, Micro & Optoelectronics Gas-Chem-Bio Sensors & Systems, at GE Research; Ed Stetter is General Manager at SPEC Sensors, LLC; Ryotaro Sakauchi, is Senior Manager of Business Development at Bosch Sensortec; Merry Smith is a Product Manager and Senior Scientist at C2Sense, Inc.; and Sreeni D. Rao is Senior Director of the MEMS Business Group at TDK Corporation.